Atomic Force Microscopy
AFM for the elucidation of solid state syntheses which avoid wastes
Article installed with permissions of the publisher
WWW-adaption by M. Haak
The AFM-technique provides new answers to the question of organic solid
state reactivity and it uncovers unexpected mechanistic details, which cannot
be observed by other analytical procedures. The new concept of phase
rebuilding provides comprehension of the longrange anisotropic molecular
movements on the basis of crystal packing data. Previously such movements
thought impossible and were not forseen, but they now prove essential for the
chemical reactivity. Thus a theoretical comprehension of surprisingly
efficiant waste-free techniques of syntheses is provided for the first time
and such syntheses have been developped up to the kg scale already. Examples
for gas/solid- and solid/solid-reactions have been chosen from various
branches of organic chemistry. All of them run uniformly and quantitatively
and are clearly superior to the corresponding liquid phase reactions with
their waste problems. Therfore gas/solid reactions are not only able to
detoxicate atmospheric air or exhaust gases but are particularly important in
view of their potential as primary action for the avoidance of wastes in
industrial chemical production. Both gas/solid- und solid/solid-reactions are particularly
simple and cost-effective because they avoid solvents and liquid phases.
AFM helps in finding systematizing and predicting further examples by indicating
the reasons for the success of these synthese techniques for the future.
Introduction
Atomic Force Microscopy (AFM) is a very young surface technique. It was
invented in 1986 [1] and unlike the 5 years older Scanning
Tunneling Microscopy (SFM) it is able to image electrically non-conducting surfaces as
well. In favourable cases AFM may resolve single molecules (more rarely single
atoms) laterally and vertically [2]. However while the recording of organic
molecular steps poses no problems to AFM [3], the imaging of individual atoms
is more practical with STM for technical reasons. The longer known STM scans
constant tunneling currents, whereas the AFM scans essentially van-der-Waals
forces. Meanwhile about 30 different SXM-techniques are available, however,
their description would exceed the scope of this contribution.
Imaging of individual atoms is epoch-making. For applied AFM it is important
to scan in the nm- and µm-range if the surface structures happen to occupy
that range. Both physical properties and chemical reactions may depend on
submicroscopic surface structures. The requirements for the scanning of large,
steep, densely packed, deeply penetrating nanostructures with AFM have been
summarized [4]. In Ref.[4] is also shown
how changes of the surface during
the scanning are avoided, how artefacts are recognized, and how the images
look like if nanoliquids form at the surface or if the limits inherent to the
AFM techniques are surpassed, an example being the imaging of membrane pores.
Waste-free solid state syntheses which do not use solvents are the future
production techniques, undoubtedly. Solid-state photolyses are longer used
already [3] however, their large scale technical use poses practical problems.
Much more interesting are gas/solid- [5] and
solid/solid-syntheses [6]. Both of these deserve puplic support and publicity.
The development of such techniques was hampered, because incomplete theories of the so called
topochemistry [7] were not able to "understand" the occurrance of such
reactions. Despite the fact that most reactions of the new techniques for
syntheses proceed quantitatively and avoid all kinds of waste (several
examples have been performed in the kg scale) the opinion still prevails,
that one should rather dissolve the reactants even at the expense of usually
lowered yield and unnecessary problems with waste. Therefor it is very
favorable that the investigation of organic gas/solid- [5] and organic
solid/solid-reaktions [8] with the AFM uncovered unexpected new theoretical
foundations. Thus primary actions may now be accepted which avoid waste
problems because there is not only an experimental basis but also a theoretical one.
Atomic Force Microscopy
An atomic force microscope [1] has a surprisingly simple
design and it does
not require unusually high investments for its purchase and use, at least as
long as ambient conditions (1 bar at RT) are prefered, which in any care are
favorable for handling volatile organic crystals. Figure 1 shows the basic
setup for the most used commercial version of an AFM.
A tip, usually made of silica nitride in 4-sided pyramidal form
(Si3N4-Standard tip, radius 20-50 mm, apex angle 45°,
height 2.8 µm, base 4x4 µm) is connected to a fixed substrate on a cantilever with a very low
spring constant (typically 0.1 N/m). The tip is approached to the surface
until the van-der-Waals attractive forces are 10-6-10-9 N
(in atomic resolution experiments the approach most be closer, down into the repulsive
region, and the tip must have an atomic end). The solid surface is then
scanned under the tip by mean of a XYZ piezo drive with the recording of
about 400x400 points or even more of them.
The constant height regulation during the scan is controlled by a feetback
loop of high dynamics from a divided photodiode, because the tip is not
supposed to hit any obstacles which may be present on the surface. That
feature is reached by a laser beam which is focussed to the back of the
gold-coated side of the cantilever just opposite to the side of the tip. From
there the laser beam is reflected to a mirror which guides it to the split
photodiode. Every change in height is recognized and immediately compensated
for by the Z-piezo. A surface topography is created. The feedback loop can be
based on constant heigh or on constant force between the tip und the surface.
Exhausting imaging software packages including drivers for suitable printers are
available for all commercial AFM instruments. In this work a NanoScope II AFM
from Digital Instruments has been used with NanoScope III software for the
imaging and printing with a color video printer. Further details are described
in the publications [3-5] and [8-11].
Phase Rebuilding in Solid State Reactions
The topochemical principle [7] has no answer to gas/solid- and solid/solid
reactivity, because it believes that it has to consider only those processes,
which are presumed to occur with minimal atomic molecular movements in the
range of less than 4.2 Å . However, AFM analysis shows that, with the
only exception of extremly rare topotactic photoreactions [3], all chemical
crystal reactions require long range anisotropic molecular movements over
several or up to hundreds of nm. Those movements are well directed vertically
and/or laterally, the reactions working themselves from the surface down
into the crystal. Additionally, those movements depend on the crystallographic
face wich proves that they are guided by the initial crystal lattice even in
the cases which involve movements over distances of several hundred nm.
The molecular movements create characteristic surface structures.
Right now 8 different geometric types of them are known [3-5,10]. If no
molecular movement is possible due to particular features of the crystal
packing no chemical reaction does ccur. This is also true if the distances
of potentially reactive centers are very short (<4.2 Å ) and their orientations
are favourable. Also numerous positive exceptions to topochemistry (solid state
reaction despite large distances of up to 10 Å ) are now easily
comprehended [3].
The experimental results enforce the introduction of the new principle of
"phase rebuilding" [3-5, 9-11]. That principle stresses the need for
immediate accommodation of new molecules with the crystal lattice present,
after their formation in solid state chemical reactions. As the product
molecules have shapes that differ from those of the starting molecules, strain
is created which has to be released by movements along the easiest ways the
crystal packing allows for (face dependancy). Only if chemical transformation
has increased so largely that the distorted original lattice cannot be retained
for thermodynamic reasons, a phase transformation may occur in which mixed
crystals of starting material (low amounts of product) transform into mixed
crystals of product (with low amounts of starting material). Thus, it is
frequently observed that initially formed surface features disappear on
prolonged reaction at the expense of new features which may be considerably
larger.
If crystal data are available it proves possible in all studied examples to
correlate the geometric type of surface feature due to phase rebuilding with
the molecular packing. It is thus secure to conclude that anisotropic molecular movements
are the decisive criterion for solid state reactivity, though in addition to
thermodynamic feasibility, of course.
Gas/Solid-Syntheses Using Acetone Vapor
Acetone is a low boiling volatile solvent of multiple use in research and
production. Its vapors in exhaust air have to be removed down under the
detection limit. Gas/solid reactions which form valuable and usable products
(instead of waste, subject to disposal) are well suited for that purpose. Such a
technique has been recently realized on the 200 g scale [9]. In that
procedure hydroxylaminiumphosphate and K2HPO4*H2O are reacted with highly
diluted acetone vapor at 80 °C and a flow rate of 100 ml/min. The Reaction
runs to completion, water and the valuable products acetoneoxime and KH2P04
are formed. That procedure is at the same time the most environmentally
friendly synthesis of acetoneoxime, which can, of course, also be performed with
nearly saturated acetone vapor in chemical production or for student
classes. From the numerous preparatively useful gas/solid reactions of
acetone with primary amine reagents and aminothiols we discuss here its
reaction with hydroxylamine hydrochloride to give acetoneoxime hydrochloride.
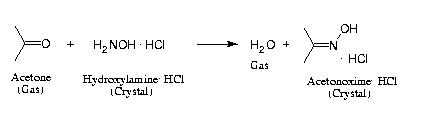
Reaction Scheme 1
The AFM-investigation with single crystals of hydoxylamine hydrochloride
(from methanol, main face) shows, that steep surface features form in moist
air (18 °C, rel. humidity 75%) within 200 min. Those are solid surface hydrates
which react considerably faster with gaseous acetone than do unhydrated
surfaces of the same reactant. Thus, it is clear, that the water of reaction
acts autocatalytically if the reaction proceeds from the surface region into
the interior of the crystal.
Figure 2: AFM surface of hydroxylaminehydrochloride;
a: main surface after 20 min manipulation in moist air;
b: after 200 min hydration in moist air;
d: same as b but inverted image;
c: after 2 min application of diluted acetone vapor on the surface hydrate
of hydroxylaminehydrochloride.
Figure 2 shows both the hydration and the reaction of the surface hydrate
with acetone vapor at short exposure. It can be seen, that the hydration
forms very steep surface structures with heights of more than 1 µm. These
features do not exhibit any asymmetries and are thus free of artefacts, at
least in their upper parts [4]. However,the structures stand so densely
(about one per µm2), that the rather large standard tip cannot reach the
ground without its pyramidal faces being touched by the features. That
circumstance is not seen in Figure 2b (and even less so in 2D-projections of
it), but very clearly in the inverted perspective image (Figure 2d) which is
most easily obtainable. In the inverted image the tops are pointing down and
the depths are pointing up. The sharp edges which appear show that the
pyramidal faces and the edges of the tip had contact with the cones (or even
columns ?) on the surface while it tried to descend into the depths. Sometimes,
if there was simultaneous contact from all sides a true image of the
pyramidal tip is seen in Figure 2d (therein enlargement of the Z-scale!). It
is to be concluded, that the surface features in Figure 2b are both taller
and higher than can be traced with a standard AFM-tip. The advantages of
perspective imaging over 2D-projections (which cannot image such tip/surface
convolutes) are evident.
The reactions of the (hydrated) hydroxylaminehydrochloride surface with acetone
vapor leads to massive structures which are more than 4 µm high and exhibit
the heights and valleys type (Figure 2c). The molecular movements that have
occurred are very far-reaching when compared with molecular dimensions. The
gas/solid reaction is unusually effective and the stability of the AFM-images
during more than 10 consecutive scans proves that no liquid or nanoliquid
phases occur [4].
Gas/Solid-Reactions with Amines
Gaseous ammonia and gaseous amines are formed in world-wide agriculture and
biological metabolism. They are responsible for new damages to forests by
their overfertilizing when entering through the atmosphere. Therefor,
gas/solid reactions of these species are of particular importance for
atmospheric chemistry and the development of detoxification strategies. In
addition to that it is also of importance to create new synthetic techniques
which avoid all kinds of waste [10]. Numerous systems have been realized. From
these the addition of methylamine to crystalline thiohydantoine with ring
opening has been chosen for analysis with the AFM.
Reaction Scheme 2
(N-Methylacetamidomethyl)thiourea is obtained quantitatively and its
waste-free formation has synthetic potential. The AFM-scans in Figure 3 show
a smooth natural (110)-surface which separates into heights and valleys after
treatment with methylamine gas. The direction of preference is along the
crystallographic cleavage plane present in the original crystal lattice.
Again, we see long range molecular movements as a consequence of phase
rebuilding and there is a clearcut relation to the crystal packing.
Figure 3: Natural (110)-AFM-surface of thiohydantoine;
a: fresh;
b: after repeated treatment with gaseous methylamine
Gas/Solid Syntheses with Nitrogen Dioxid
Nitrogen dioxid is a toxic gas from (automotive) firings which spoils the
environment and which cannot be readily controlled, yet. It is a free radical
and has a high reactivity which can be used in numerous gas/solid syntheses
which, unlike common solution reactions, proceed quantitatively and
waste-free [11].
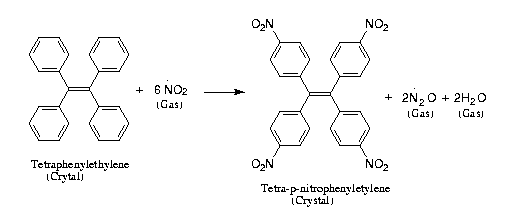
Reaction Scheme 3
The quantitative tetranitration of tetraphenylethylene is quite spectacular
(NO is oxidized with O2 to NO2 and thus recycled). Conventional techniques
of synthesis yield 65-70 % yield at most (under optimized conditions) from a
highly corrosive nitric acid solution after enormous efforts of purification
and with severe problems in waste disposal. The AFM scans in Figure 4 show,
that cyclic craters with nicely shaped walls of diverse size form upon
phase rebuilding in this reaction. That is seen both in the perspective and in
the 2D-projection (Figure 4b, d).
Figure 4: AFM-surface of tetraphenylethylene;
a: fresh natural (10-1)-surface;
b/d: after 10 min exposure to 0.2 bar of NO2;
c: after 20 min exposure to 0.2 bar of NO2;
Upon longer exponere to NO2 gas the circumwalled craters disappear, no doubt, as a consequence of phase
transformation into the product lattice. The initially formed cyclic
structures are again comprehended by correlation with the crystal structure
of the starting material [11]. The tetraphenylethylene molecules which stand
vertical under (10-1) have a limited possibility for partial turns around
their long axis. They use it if they grow thicker upon nitration. By doing
that they collide with next row molecules which then turn in opposite
direction and provoke a third and then a forth molecule to the corresponding
turns with finally filling the hole left from the first of these molecules.
Thus, a concentric process leaving a hole at its center is described. After
such initiation the growing molecules (consecutive nitrations) move upward
and present their bottom p-positions for nitration with NO2 gas. The crater
diameter is enhanced by participation of neighbouring rows of molecules and
those processes proceed until the original lattice breaks down [11]. Again,
the crystal structure dictates the long range anisotropic molecular movements
during phase rebuilding.
Contrary to solution diazotations, gas/solid diazotation may proceed
waste-free and allow elegant applications in syntheses [12]. The solid
state diazotation of sulfanilic acid to give its diazonium salt, which is
needed for the synthesis of widely used pH-indicator methylorange (via azo
coupling with N,N-dimethylaniline) provides particularly impressive
AFM-images.
Reaction Scheme 4
Both anhydrous sulfanilic acid or its monohydrate, which forms monoclinic
prisms with 1-3 mm long edges, diazotize quantitatively under the action of
gasous NO2. The solid product crystals may be ground for IR-pellets etc. They
are however, as are other solid diazonium salts, explosive if heavily hit
with a hammer on an anvil. The AFM-investigation on the (010) pinacoid of
sulfanilic acid monohydrates (at 22°C in the air with a rel. humidity of 41%)
is shown in Figure 5.
Figure 5. (010)-AFM surface of sulfanilic acid monohydrate;
a: fresh and barely effloresced;
b/d: island formation after short diazotation with NO2-gas;
c: after advanced diazotation by several treatments with NO2.
The initial surface in Figure 5a shows traces of efflorescence which may be the
reason for the volcano like features. These are swallowed after short
application of gaseous NO2 to the surface in air. Flat islands which may
enclose little lakes are formed (Figure 5b, d). The characteristic island
features are still recognizable after multiple application of NO2 (Figure 5c).
However, the advanced height-growth is less uniform. A more roughened height
scenery with embraced depths is optained. The formation of islands [10] is
easily derived from the crystal structure of sulfanilic acid hydrate [13],
which is depicted in Figure 6.
Figure 6. Space filling stereoscopic representation of the molecular packing
of sulfanilic acid monohydrate;
top: view on the (010)-face showing hydrogen bridges between NH3+ and SO3- groups
with additional H-bridging by H2O molecules;
bottom: view on (100) which is orthogonal to (010) showing how the molecules
alternate in their orientation and how they stand long-elongated under
the (010) face which is on top;
N: grid; S: shaded; O at S: circles; O of the water: dense circles.
The molecules stand vertically under (010) and their orientation alternates.
Thus, a maximum of hydrogen bridges is obtained and the water molecules add
further H-bridging. While the upmost layer on (010) is open for attack of the
NH2 groups by NO2 of half of the molecules (Figure 6, top), the proceeding of
the reaction into the crystal requires, that long elongated molecules exit
from their H-bonded lattice positions and move upward in order to make the
amino groups available for diazotation which pointed downward from the first
layer and upward from the second layer etc.
Clearly the reaction is hindered by this particular packing. Therefore the
reaction spreads around the initial successfull invasions into row 2 etc. and
thus from flat islands. 2 planes with a distance of 15 nm are present in Figure 5b/d.
Solid/Solid-Reactions
Waste-free syntheses which succeed by simply mixing of crystalline reactants
are particularly attractive for saving ressources [6]. The first
AFM-investigation of such a reaction concern the quantitative pinacol
rearrangement (elimination of water with rearrangement) of benzopinacol [8].
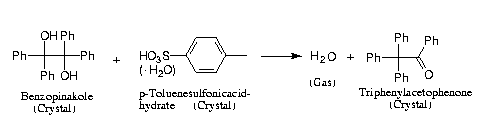
Reaction Scheme 5
A small crystal of p-toluenesulfonic acid hydrate is placed on the (100)-face
of a larger single crystal of benzopinacol for the AFM-investigation. The
large crystal is scanned at different distances from the edges of the small
one. At 0.5 mm distance (Figure 7a) a moderately stepped surface image is
obtained. The same is true at 0.1, 1 and 2 mm distance. Here and in the
subsequent AFM-scans it does not make any difference if measurement is done
along the b-axis or at 90°, 60° or 45° to it. Also higher terrasse steps are
found in larger scale 10 µm-scans [8].
Figure 7: AFM-surface of benzopinacol (100);
a: fresh in a distance of 0.5 mm from an onlaying crystal of p-toluenesulfonic acid monohydrate
b/d: after 12 h at 50 °C, at a distance of 0.5 mm;
c: after 12 h at 50 °C, at a distance of 0.1 mm;
The crystals are heated to 50°C for 12 h to initiate the reaction. They are
remounted to the AFM and then scanned. One obtaines well-formed craters in
0.5 mm distance from the proton source (Figure 7b,d). Those are 5 mm deep and
150 mm wide. Importantly the reaction has much more proceeded it 0.1 mm
distance (Figure 7c). The craters found there are about 11 nm deep and are
mostly circumwalled with heights of typically 4 nm. Only at distances of more
than 2 mm one finds surface images similar to the one in Figure 7a.
The distance dependent reaction and crater formation can be understand again
in terms of crystal data [8]. Here catalytic quantities of protons move over
2 mm from the small acid crystal and produce a charge separation, causing the
crystals to stick together firmly. Mechanistically the protons combine with
OH groups at the front side of molecular layers and water is liberated.
Therefor, new protons are formed at the backside of cations after their
rearrangement to yield triphenylacetophenone. These newly formed protons
react with the frontside OH groups of the next layer etc. [8].
Interestingly, the morphologically dominant (001)-face does not react
correspondingly, according to the AFM investigation. That surface remains
unchanged after the same treatment and the acid crystal does not stick to
that surface of benzopinacol. The reason for that is to be found in the
orientation of the OH groups in the upmost layer of benzopinacol. On the
(001)-face the hydroxyl-H (but not the free electron pairs) point outward.
Therefor, no protonation can occur, inasmuch as there is also steric hindrance
[8]. Conversely, on the reactive (100)-face, the free electron pairs are
pointing outward and may thus be protonated [8].
This first mechanistic investigation of a crystal/crystal-reaction shows the
potential of AFM and indicates a mechanistic understanding of the many further
waste-free solid/solid-reactions which occur in most diverse types [6].
Literature
[1] G. Binning, C. Quate, C. Gerber: Atomic force microscope,
Phys. Rev. Lett. 56 (1986) 930.
[2] J. Frommer: Scanning Tunneling and Force Microscopy in
Organic Chemistry, Angew. Chem. Int. Ed. Engl. 104 (1992) 1324.
[3] G. Kaupp: AFM and STM in photochemistry including photon
tunneling, Advances in Photochemistry 19 (1995) 119.
[4] G. Kaupp, J. Schmeyers, U. Pogodda, M. Haak, T. Marquardt, M. Plagmann:
AFM for the imaging of large and steep submicroscopic features,
artifacts and scraping with asymmetric cantilever tips,
Thin Solid Films, SXM1 special issue, 1995, in press.
[5] G. Kaupp: Atomic force microscopy in organic gas/solid-reactions:
How do the new phases build up?, Mol. Cryst. Liq. Cryst. 211 (1992) 1, cit. lit.
[6] F. Toda in Y Ohashi (Hrsg.): Reactivity in Molecular Crystals,
Kap. 4: Solid-to-solid organic reactions, Kodansha/VCH, Tokyo/Weinheim, 1993, S. 177.
[7] M. D. Cohen, G. M. J. Schmidt, F. I. Sonntag: J. Chem. Soc.
(1964) 2000; G. M. J. Schmidt: ibid. (1964) 2014;
M. D. Cohen: Angew. Chem. 87 (1975) 439; Mol. Cryst. Liq. Cryst. 50 (1979) 1.
[8] G. Kaupp, M. Haak, F. Toda: Atomic force microscopy and solid
state rearrangement of benzopinacol,
J. Phys. Org. Chem. S 95 H 12, in press.
[9] G. Kaupp, U. Pogodda, J. Schmeyers: Gas/solid-reactions with
acetone, Chem. Ber. 127 (1994) 2249; cit. lit.
[10] G. Kaupp, J. Schmeyers: Gas/Solid-Reactions of Aliphatic Amines
with Thiohydantoines: Atomic Force Microscopy and New Mechanisms,
Angew. Chem. Int. Ed. Engl. 105 (1993) 1656.
[11] G. Kaupp, J. Schmeyers: Gas/solid-reactions with nitrogen
dioxide, J. Org. Chem. 60 (1995) Heft 16, in press.
[12] A. Herrmann: Diploma Thesis, University of Oldenburg, 1995.
[13] A. I. M. Rae, E. N. Maslen: The crystal structure of
sulfanilic acid mono-hydrate, Acta Cryst. 15 (1962) 1285.
Supplementary Material:
A comparable illustration about the use of AFM elucidating mechanisms of crystal photolyses can be found in
G. Kaupp: Fachzeitschrift für das Laboratorium GIT 37 (1993) 284-294 und 581-586.
haak@kaupp.chemie.uni-oldenburg.de